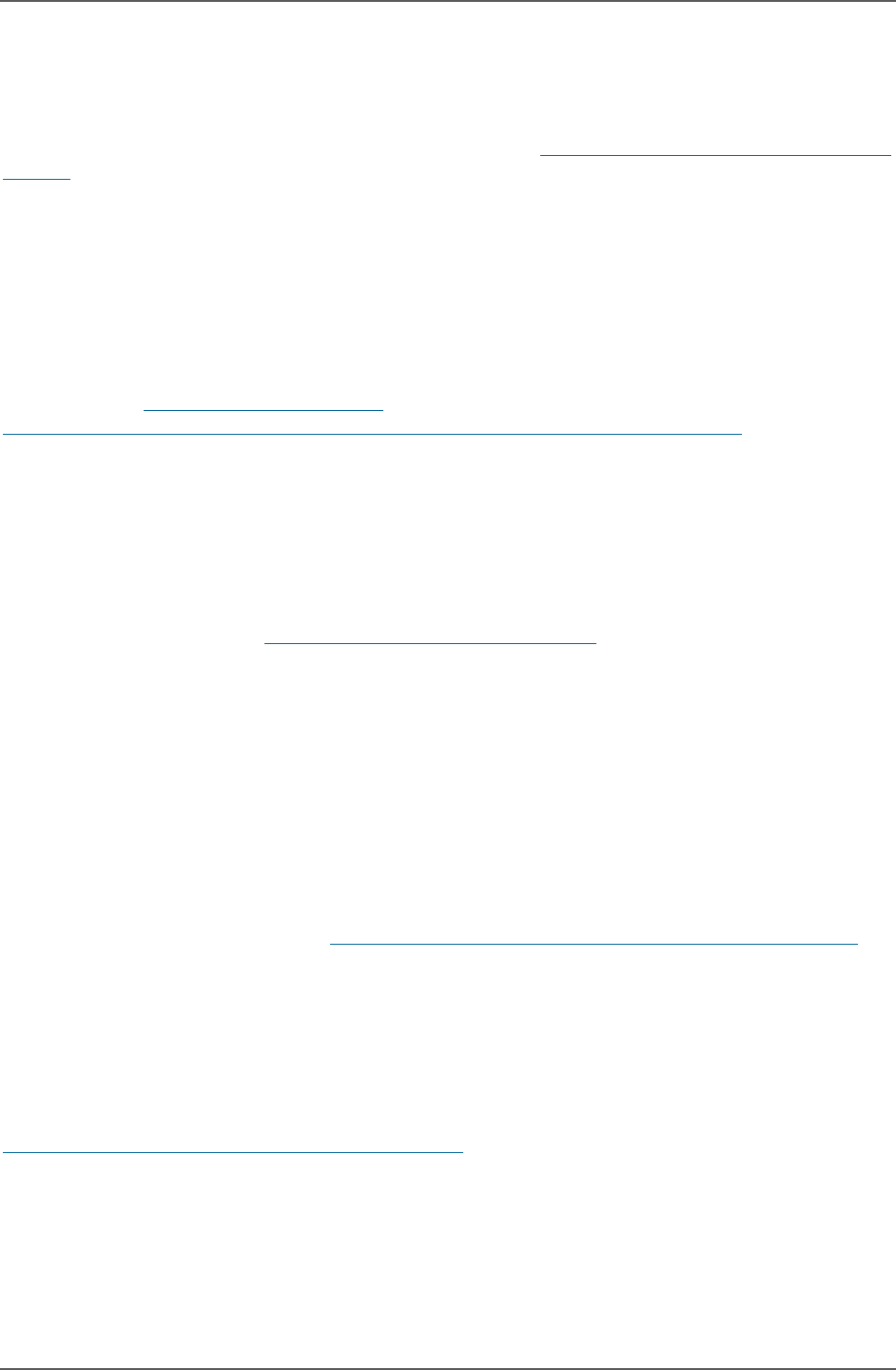
FEMA Ecosystem Service Value Updates
185
199
National Recreation and Park Association (NRPA). 2017. Resource Guide for Planning, Designing and Implementing
Green Infrastructure in Parks. NRPA, Ashburn, Virginia. Available online at: https://www.nrpa.org/siteassets/gupc-resource-
guide.pdf
200
Bockarjova, M., Botzen, W.J., Koetse, M.J. 2020. Economic valuation of green and blue nature in cities: A meta-analysis.
Ecological Economics 169: 106480.
201
Qiu, Z., Prato, T., Boehm, G. 2006. Economic Valuation of Riparian Buffer and Open Space in a Suburban Watershed.
Journal of the American Resources Association 42(6): 1583–1596.
202
Interagency Working Group on Social Cost of Greenhouse Gases. 2021. Technical Support Document: Social Cost of
Carbon, Methane, and Nitrous Oxide Interim Estimates under Executive Order 13990. White House, Washington, DC.
Available online at: https://www.whitehouse.gov/wp-
content/uploads/2021/02/TechnicalSupportDocument_SocialCostofCarbonMethaneNitrousOxide.pdf
203
Lu, X., Kicklighter, D.W., Melillo, J.M., Reilly, J.M., Xu, L. 2015. Land carbon sequestration within the conterminous
United States: Regional‐and state‐level analyses. Journal of Geophysical Research: Biogeosciences 120(2): 379-398.
204
Liu, S., Liu, J., Young, C.J., Werner, J.M., Wu, Y., Li, Z., Dahal, D., Oeding, J., Schmidt, G., Sohl, T.L., Hawbaker, T.J.,
Sleeter, B.M. 2012. Chapter 5: Baseline Carbon Storage, Carbon Sequestration, and Greenhouse-Gas Fluxes in Terrestrial
Ecosystems of the Western United States. In: Zhu, Z., Reed, B.C. (eds.). Baseline and Projected Future Carbon Storage and
Greenhouse-Gas Fluxes in Ecosystems of the Western United States (p. 45-63). U.S. Geological Survey Professional Paper,
Reston, Virginia. Available online at: https://pubs.er.usgs.gov/publication/pp1797
205
DeLonge, M.S., Ryals, R., Silver, W. 2013. A Lifecycle Model to Evaluate Carbon Sequestration Potential and
Greenhouse Gas Dynamics of Managed Grasslands. Ecosystems 16: 962-979.
206
Ryals, R., Silver, W.L. 2013. Effects of organic matter amendments on net primary productivity and greenhouse gas
emissions in annual grasslands. Ecological Applications 23: 46-59.
207
Schuman, G.E., Janzen H.H., Herrick J.E. 2002. Soil carbon dynamics and potential carbon sequestration by rangelands.
Environmental Pollution 116: 391-396.
208
Smith, J.E., Heath, L.S., Skog, K.E., Birdsey, R.A. 2006. Methods for calculating forest ecosystem and harvested carbon
with standard estimates for forest types of the United States. USDA Forest Service Northeastern Research Station,
Amherst, Massachusetts. Available online at: https://www.fs.fed.us/ecosystemservices/pdf/estimates-forest-types.pdf
209
Pimentel, D., Harvey, C., Resosudarmo, P., Sinclair, K., Kurz, D., McNair, M., Crist, S., Shpritz, L., Fitton, L., Saffouri, R.,
Blair, R. 1995. Environmental and Economic Costs of Soil Erosion and Conservation Benefits. Science 267: 1117-1123.
210
Bockarjova, M., Botzen, W.J., Koetse, M.J. 2020. Economic valuation of green and blue nature in cities: A meta-analysis.
Ecological Economics 169: 106480.
211
U.S. Census Bureau. Nd. QuickFacts: United States. Available online at:
https://www.census.gov/quickfacts/fact/table/US/PST045219
212
Pimentel D., Wilson, C., McCullum, C., Huang, R., Dwen, P., Flack, J., Tran, Q., Saltman, T., Cliff, B. 1997. Economic and
Environmental Benefits of Biodiversity. BioScience 47(11): 747-757.